Potential Role of Betel Leaf (Piper betle L.) Water Extract as Antibacterial Escherichia coli Through Inhibition of β-Ketoacyl-[Acyl Carrier Protein] Synthase I
http://www.doi.org/10.26538/tjnpr/v6i11.10
Keywords:
Herbal medicine, Fatty acid, FabB, Competitive inhibitor, Chronic woundAbstract
The exploration of bioactive compounds from herbal plants as inhibitors of fatty acid synthesis without adverse side effects is still the focus of the discovery and development of antibacterial drugs, especially to fight infections in chronic wounds. This study aimed to investigate the biological function of the bioactive compound of Piper betle L. leaf extract in inhibiting the target protein of Escherichia coli. 3D structure of the bioactive compound and thiolactomycin (as a positive control) were downloaded from PubChem. The Protein Data Bank database was used to retrieve the 3D structure of β-Ketoacyl-[Acyl Carrier Protein] Synthase I (FabB). Prediction of the active site of FabB was predicted using the Molegro Virtual Docker 5.0 program. Molecular interactions of the compound and target protein were analyzed using Molegro virtual docking version 5, superimposed using PyMol version 2.2, and visualized using the Discovery Studio program version 21.1.1. The results showed that the five bioactive compounds of Piper betle L. (eugenol, catechin, caffeic acid, quercetin, and ascorbic acid) could bind to the active site of FabB. All compounds were found to bind to the residues ALA271, PRO272 and HIS298. The catechin-FabB complex showed the lowest binding energy. This finding indicates that the Piper betle L. leaf extract's bioactive compound may inhibit the target protein of E. coli β - Ketoacyl-[Acyl Carrier Protein] Synthase I (FabB), which leads to the
control of bacterial infection in chronic wounds at the cellular level.
References
Negut I, Grumezescu V, Grumezescu AM. Treatment strategies for infected wounds. Molecules 2018; 23:1–23.
Trinh XT, Long N Van, Van Anh LT, Nga PT, Giang NN, Chien PN. A Comprehensive Review of Natural Compounds for Wound Healing: Targeting Bioactivity Perspective. Int J Mol Sci. 2022; 23.
Raziyeva K, Kim Y, Zharkinbekov Z, Kassymbek K, Jimi S, Saparov A. Immunology of acute and chronic wound healing. Biomolecules. 2021; 11:1–25.
Garraud O, Hozzein WN, Badr G. Wound healing: Time to look for intelligent, ‘natural’ immunological approaches? BMC Immunol. 2017; 18:40.
Murphy PS and Evans GRD. Advances in Wound Healing: A Review of Current Wound Healing Products. Plast Surg Int. 2012; 2012:1–8.
Han G and Ceilley R. Chronic Wound Healing: A Review of Current Management and Treatments. Adv Ther. 2017; 34:599–610.
Tom IM, Ibrahim MM, Umoru AM, Umar JB, Bukar MA, Haruna AB. Infection of Wounds by Potential Bacterial Pathogens and Their Resistogram. OALib. 2019; 06:1–13.
Ding X, Tang Q, Xu Z, Xu Y, Zhang H, Zheng D. Challenges and innovations in treating chronic and acute wound infections: from basic science to clinical practice. Burn Trauma 2022; 10.
Wilgus TA, Roy S, McDaniel JC. Neutrophils and Wound Repair: Positive Actions and Negative Reactions. Adv Wound Care. 2013; 2:379–388.
Liu YF, Ni PW, Huang Y, Xie T. Therapeutic strategies for chronic wound infection. Chinese J Traumatol - English Ed 2022; 25:11–16.
Puca V, Marulli RZ, Grande R, Vitale I, Niro A, Molinaro G. Microbial species isolated from infected wounds and antimicrobial resistance analysis: Data emerging from a threeyears retrospective study. Antibiotics 2021; 10.
de Prost N, Lipman J, Mimoz O. Therapeutic targets in necrotizing soft tissue infections. Intensive Care Med 2017; 43:1717–1719.
De Francesco F, Riccio M, Jimi S. Contribution of Topical Agents such as Hyaluronic Acid and Silver Sulfadiazine to Wound Healing and Management of Bacterial Biofilm. Med. 2022; 58.
Mihai MM, Preda M, Lungu I, Gestal MC, Popa MI, Holban AM. Nanocoatings for chronic wound repair—modulation of microbial colonization and biofilm formation. Int J Mol Sci. 2018; 19.
Perdana I, Suharto S, Ramayanti ED, Yunalia M, Ulfa N. Betel Leaf Extract’s Effect (piper batle Linn) on The Healing Process of Incision Wounds in Rats (Rattus Norvegicus). J Pendidik Keperawatan Indones 2021; 7:123–128.
Lubis RR, Marlisa, Wahyuni DD. Antibacterial activity of betle leaf (piper betle l.) extract on inhibiting Staphylococcus aureus in conjunctivitis patient. Am J Clin Exp Immunol 2020; 9:1–5.
Ghazali NA, Elmy A, Yuen LC, Sani NZ, Das S, Suhaimi F. Piper betel leaves induces wound healing activity via proliferation of fibroblasts and reducing 11β hydroxysteriod dehydrogenase-1 expression in diabetic rat. J Ayurveda Integr Med 2016; 7:198–208.
Lien LT, Tho NT, Ha DM, Hang PL, Nghia PT, Thang ND. Influence of phytochemicals in piper betle linn leaf extract on wound healing. Burn Trauma. 2015; 3:1–8.
Purba RAP and Paengkoum P. Bioanalytical HPLC method of Piper Betle L. for quantifying phenolic compound, water-soluble vitamin, and essential oil in five different solvent extracts. J Appl Pharm Sci. 2019; 9:33–39.
Nayaka NMDMW, Sasadara MMV, Sanjaya DA, Yuda PESK, Dewi NLKAA, Cahyaningsih E. Piper betle (L): Recent review of antibacterial and antifungal properties, safety profiles, and commercial applications. Molecules 2021; 26:1–21.
Surjowardojo P, Setyowati E, Ambarwati I. Antibacterial Effects of Green Betel (Piper betle Linn.) Leaf Against. AGRIVITA J Agric Sci. 2019; 41:569–574.
Niquehat Noor and Satapathy KB. Phytochemical Screening and Antibacterial Potential of Wild Leafy Vegetable- Cayratia auriculata (Roxb.) Gamble Against Selected Enteric Pathogens. Trop J Nat Prod Res. 2021; 5:621–625.
Kassab E, Fuchs M, Haack M, Mehlmer N, Brueck TB. Engineering Escherichia coli FAB system using synthetic plant genes for the production of long chain fatty acids. Microb Cell Fact. 2019; 18:1–10.
Janßen HJ and Steinbüchel A. Fatty acid synthesis in Escherichia coli and its applications towards the production of fatty acid based biofuels. Biotechnol Biofuels 2014; 7:1–26.
Pavoncello V, Barras F, Bouveret E. Degradation of Exogenous Fatty Acids in Escherichia coli. Biomolecules 2022; 12.
Yao J and Rock CO. Bacterial fatty acid metabolism in modern antibiotic discovery. Biochim Biophys Acta - Mol Cell Biol Lipids. 2017; 1862:1300–1309.
Nanson JD, Himiari Z, Swarbrick CMD, Forwood JK. Structural Characterisation of the Beta-Ketoacyl-Acyl Carrier Protein Synthases, FabF and FabH, of Yersinia pestis. Sci Rep 2015; 5:1–13.
Price AC, Choi KH, Heath RJ, Li Z, White SW, Rock CO. Inhibition of β-ketoacyl-acyl carrier protein synthases by thiolactomycin and cerulenin: Structure and mechanism. J Biol Chem 2001; 276:6551–6559.
Bitencourt-Ferreira G and de Azevedo WF. Docking Screens for Drug Discovery. Humana Press: Ria Grande do Sul, 2019. 30 Wang Y, Ma S. Recent advances in inhibitors of bacterial fatty acid synthesis type II (FASII) system enzymes as potential antibacterial agents. ChemMedChem. 2013; 8:1589–1608.
Hiseni A, Otten LG, Arends IWCE. Identification of catalytically important residues of the carotenoid 1,2-hydratases from Rubrivivax gelatinosus and Thiocapsa roseopersicina. Appl Microbiol Biotechnol. 2016; 100:1275–1284.
Sabbagh G and Berakdar N. Docking studies of flavonoid compounds as inhibitors of β-ketoacyl acyl carrier protein synthase I (Kas I) of Escherichia coli. J Mol Graph Model. 2015; 61: 214–223.
Silva JC, Silva Pereira RL, Sampaio de Freitas T, Rocha JE, Macedo NS, de Fatima Alves Nonato C. Evaluation of antibacterial and toxicological activities of essential oil of Ocimum gratissimum L. and its major constituent eugenol. Food Biosci 2022; 50.
Ouertani A, Neifar M, Ouertani R, Masmoudi AS, Cherif A. Effectiveness of enzyme inhibitors in biomedicine and pharmacotherapy. Adv Tissue Eng Regen Med Open Access. 2019; 5:85–90.
Primiani C, DRT S, GC K, P P, MA S. Anti-Inflammatory Potentials of Elaeocarpus sphaericus Schum Fruit Compounds by Molecular Docking Approach. Trop J Nat Prod Res 2022; 6:1663–1669.
Tribudi YA, Agustin AT, Setyaningtyas DE, Gusmalawati D. Bioactive Compound Profile and Biological Modeling Reveals the Potential Role of Purified Methanolic Extract of Sweet Flag (Acorus calamus L.) in Inhibiting the Dengue Virus (DENV) NS3 Protease-Helicase. Indones J Chem. 2022; 22:331–341.
Agustin AT, Safitri A, Fatchiyah F. An in Silico Approach Reveals the Potential Function of Cyanidin-3-o-glucoside of Red Rice in Inhibiting the Advanced Glycation End Products(AGES)-Receptor (RAGE) Signaling Pathway. Acta Inform Medica 2020; 28: 170–179.
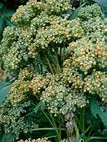
Published
How to Cite
Issue
Section
License

This work is licensed under a Creative Commons Attribution-NonCommercial-NoDerivatives 4.0 International License.